Trading Telescope for Supercomputer to Solve 400-Year-Old Solar Mystery
Since the early 17th Century, when Galileo used a telescope he made himself to observe sunspots, astronomers have been grappling with the same question: Why do sunspot counts swell and dip on a 11-year cycle?
Sunspots are dark spots on the Sun’s surface that multiply when the Sun becomes more active. When you express sunspot observation data in graph form by placing a dot for each sunspot according to its location, or solar latitude (Y-axis), and time (X-axis), you will see butterfly-shaped clusters of dots in 11-year intervals.
While it’s clear from historical data that solar activity is cyclical, the mechanism behind it has been a mystery. But scientists may be a step closer to solving it, now that an international research group based in Japan has identified the Sun’s magnetic field formation mechanism, using a supercomputer to successfully recreate changing magnetic field patterns. This is important news to those researching auroras, as well, because the two phenomena are inherently connected; the energy that produces auroras comes from the Sun.
So, what do the solar magnetic field patterns really tell us about the Sun? And how will the research group’s creative use of supercomputer help solve the four-century-old puzzle? The research’s principal investigator will take us through their cutting-edge study about the Sun, which could also provide clues to questions surrounding auroras.
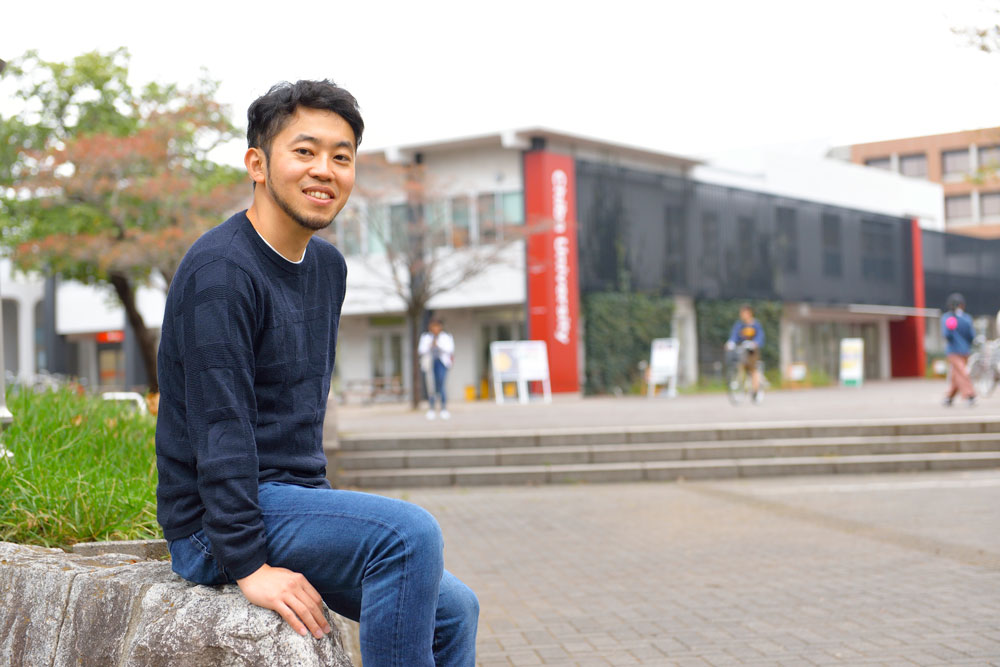
Ask the Expert: Hideyuki Hotta (Chiba University)
Hideyukai Hotta, specially appointed associate professor at Chiba University’s Graduate School of Science, Faculty of Science, conducts research on the complex mechanism of the Sun’s magnetic field formation. His paper detailing the findings from his solar activity simulations – which he had been working on since his college days – was published in the Science magazine in 2016. Dr. Hotta has served in his current position since 2015, after receiving his bachelor’s and graduate degrees from the University of Tokyo and completing his post-doctoral research at the university. His strong interest in science stems from looking at the visual presentation of Kelvin–Helmholtz instability -- hydrodynamic instability that occurs under certain circumstances -- which resembles the Japanese ukiyo-e artist Hokusai’s painting, “The Great Wave.” He was fascinated by the fact that existing patterns in science phenomena can visually emerge if one applies a math equation with the right data. At Chiba University, Dr. Hotta continued his work toward solving the mechanism behind the cyclical solar activity.
Connecting dots through math to recreate solar activity
The Sun, which is mostly made up of hydrogen and helium, is a chaotic star. Its convection zone, for example, consists of plasma — which is so hot that electrons and protons are torn apart from their respective atoms and run amok in all directions. In the meantime, some of the Sun’s colossal magnetic fields eject pent up energy, which then converts into different types of energy, such as heat and kinetic energy.
Despite all this seeming confusion, changes in solar activity mostly take place on a schedule, adhering roughly to a 11-year cycle, according to Dr. Hotta.
“In other words, if we understood how the Sun’s turbulence occurs, we could then logically explain why the cycle is 11-year,” he said.
Dr. Hotta believes it’s an achievable goal, given that the Sun’s structure and components are already well known.
“Our understanding is based on the Stellar Standard Theory. It is a stellar evolutionary theory that has been confirmed through observations,” Dr. Hotta said. “How earthquakes’ sound waves travel can also tell you a lot about the interior of the respective celestial body. The study of the Sun’s interior using this method is called helioseismology, and there has been a lot of advancement in this field in recent years. Today, the Sun’s interior can be measured with a margin of error of 0.1 percent or so. It’s safe to say we know the Sun’s inside better than that of the Earth.”
Dr. Hotta said he thought, by relying on these theories, he should be able to mathematically connect all the dots to explain the process of changing solar activity.
“Since the theory is well-established, if we cannot get the answers that we are looking for, that would mean we just need to adjust the calculation method,” Dr. Hotta said.
That’s easier said than done.
In the case of the Sun, they are smooth and flow easily.
“The smoother a plasma is, the more likely it makes many small streams,” Dr. Hotta said. “That means you need to identify and track all of them, requiring an enormous amount of calculation.”
What makes such a calculation even more difficult is the fact that the Sun’s surface is entirely different from its interior in many ways. The Sun’s surface is about 6,000 degrees Celsius, with a density of 10 to the power of minus-7 grams per cubic centimeter. In comparison, at the base of the convection zone, the temperature reaches 2 million degrees, and a density of about 0.2 grams per cubic centimeter. On the surface, thermal speed and the speed of sound are nearly equal. In the center, however, the speed of sound is about 2,000 times faster than thermal speed.
“It’s difficult to take advantage of what makes supercomputers great when things are this complicated,” Dr. Hotta said.
So, he developed a calculation method that allows him to simplify things by using an intentionally lowered speed of sound while still connecting all other dots in the right way.
He used the method to perform calculations on 96 percent of the Sun, starting from its center. And this is how in 2016, he and his team identified the Sun’s magnetic field formation patterns. (Click here for the press release issued on March 25, 2016. Dr. In a separate project, Hotta and his team completed the calculation for 99 percent of the Sun.)
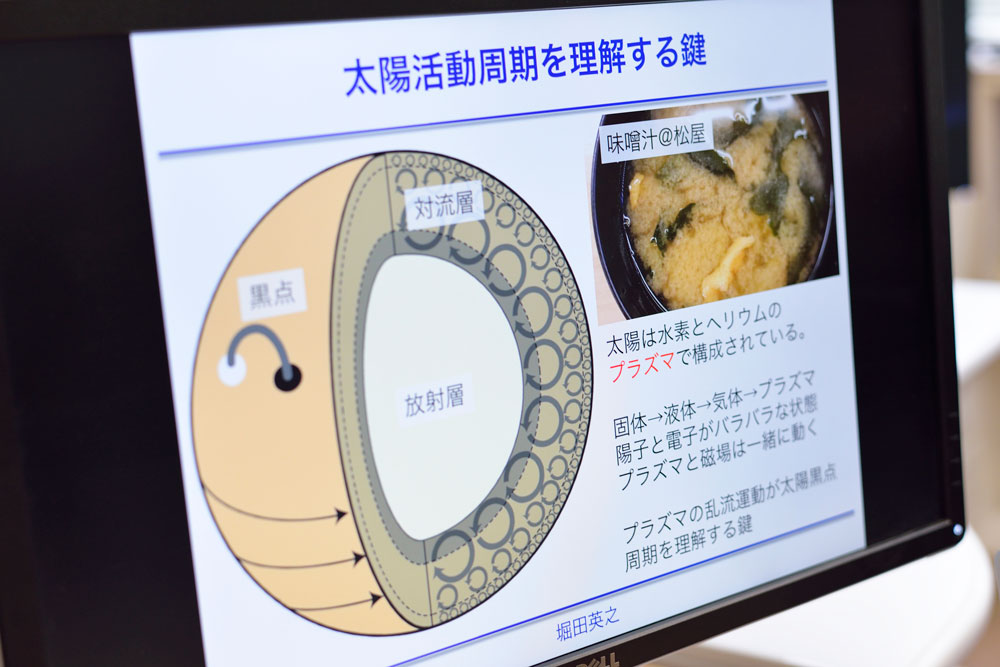
Sunspots: spots that have too much energy to get hot
So, what do we know about the Sun anyway?
The Sun produces energy in its core through nuclear fusion. Radiated energy uses the heat transfer mechanism to travel outward. It takes the heat as long as 200,000 years to penetrate the radiative zone — extending from the outer edge of the core from 20 percent of the distance to the surface and 70 percent of that distance — before eventually reaching the convection zone just above it, according to Dr. Hotta.
“The convection zone is bubbling like boiling water because there’s so much thermal convection going on. But in some parts of the zone, the magnetic fields are too strong for thermal convection to occur, causing them to stay cooler. This gives them a darker appearance in color. Those are what we call sunspots,” Dr. Hotta said.
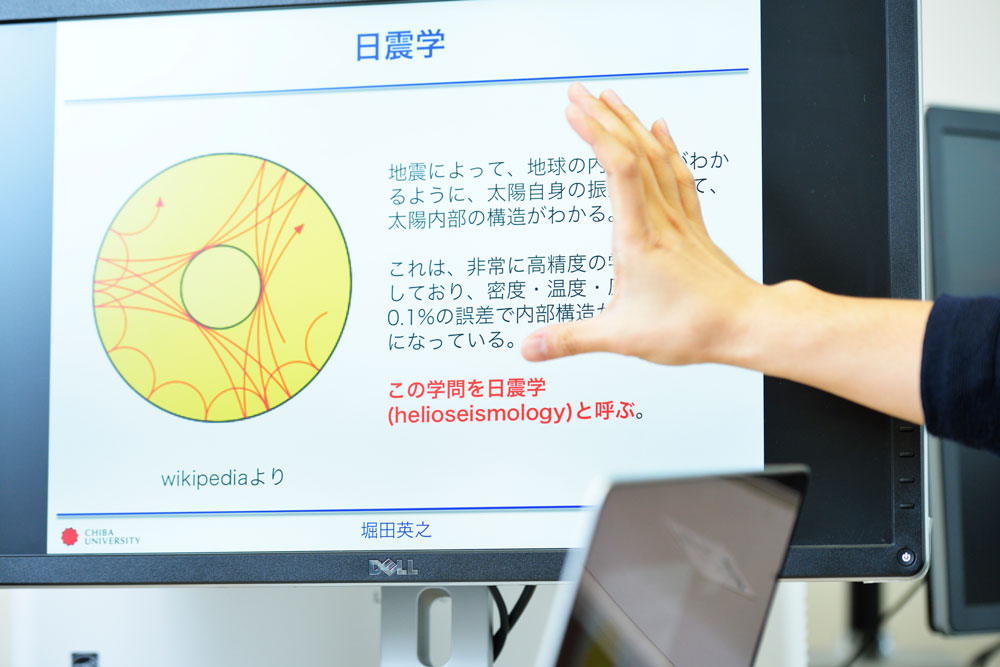
Each sunspot comes with a pair of a north and south magnetic poles, which are connected to each other with magnetic field lines. Short-circuiting of these magnetic lines causes explosive flashes of brightness, known as “flares.” In some cases, explosions have the strength equivalent to one million hydrogen bombs, setting off giant flares. When this energy reaches the Earth, it can cause auroras. One can tell that sunspots’ magnetic fields are powerful through the observation of sunlight. More sunspots emerge when solar activity picks up.
The Sun also has its north and south magnetic poles as part of its “dynamo” mechanism through which power is generated as the star spins on its axis. The Sun’s north and south poles flip every 11 years.
“But, how and why the cycle is 11 years is not understood yet. That’s solar physics’ oldest and biggest mystery,” Dr. Hotta said.
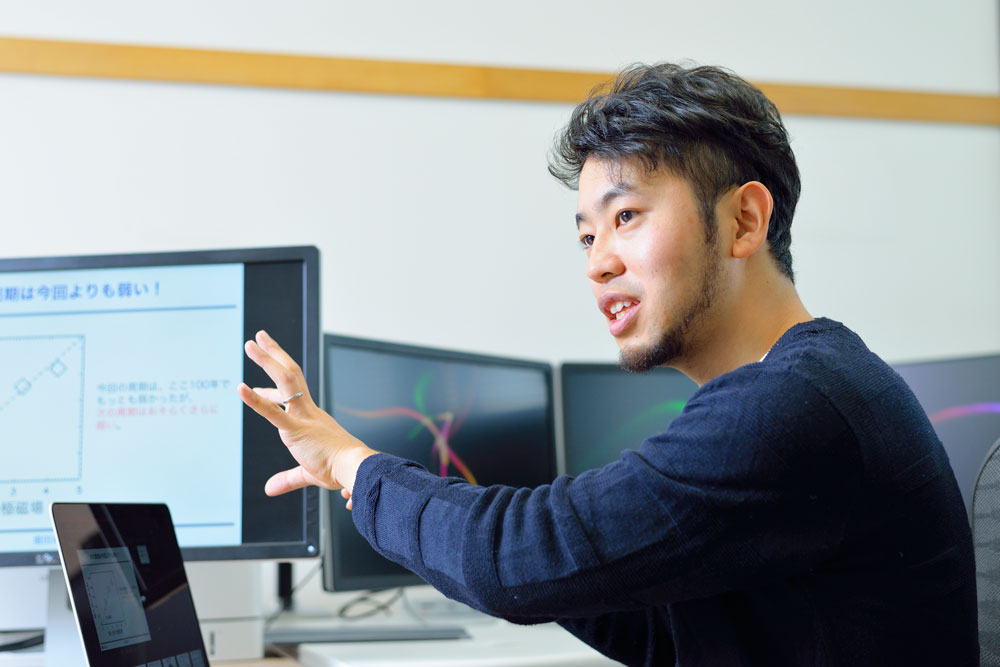
Hidden details of big solar picture
Dr. Hotta’s and his team’s success in finding the Sun’s magnetic field formation patterns is a big step forward in understanding the Sun’s 11-year cycle. Their computation method enables them to present the patterns in the highest resolution possible. Their computer simulation of solar activity is so clear and detailed that one might mistake it for satellite imagery.
The simulation shows how magnetic fields start forming in the Sun’s interior, growing outward as they swirl. “The magnetic fields form watermelon-like vertical stripes due to the Coriolis effect from the Sun’s rotation,” Dr. Hotta said.
The patterns become much more intricate on the Sun’s surface, where convective motions happen on smaller scales in a greater number.
“This calculation method made it possible, for the first time in history, to recreate solar convection from the base of the solar convection zone to near the surface all at once,” Dr. Hotta said.
“When you increase the resolution, you begin to see the magnetic fields breaking into many mini-swirls,“ he said. “As we all know, typhoons always spin in a particular direction, but the water in bathtubs doesn’t necessarily swirl down the drain in the same direction as typhoons. In other words, a celestial body’s rotation affects large swirls. When magnetic fields flow in one direction, that makes them larger and stronger.”
The small swirls recreated through the computation, however, flow in all different directions, because they are not influenced by the Sun’s rotation.
“Thus, the magnetic fields become broken down in pieces, creating a mess,” Dr. Hotta said.
Because of this, the large swirls that were visible with the use of a low-resolution calculation method became invisible in the high-resolution computation.
“The Sun’s plasmas are highly fluid. This means the higher the resolution is, the closer to reality the simulation result should be,” he said. “Magnetic fields in smaller scales quickly merge with each other and grow, producing large amounts of energy. They eventually become entangled and lose agility. At this point, they could only make large swirls. That’s what we’ve found so far.”
Dr. Hotta is using this finding as the foothold to simulate the solar activity occurring in the last 1 percent of the distance to the Sun’s surface from the core.
Finding the mechanism behind the Sun’s 11-year cycle is considered as a key to better understanding magnetohydrodynamics, which would then help further advance solar research.
“In addition, that will help us understand many other celestial bodies, as well,” Dr. Hotta said. “Observations are being conducted on a lot of different stars now, and many of them have 5 to 10-year cycles. Once we know what causes the Sun’s cycle, we could then apply the knowledge to understanding additional stars.”
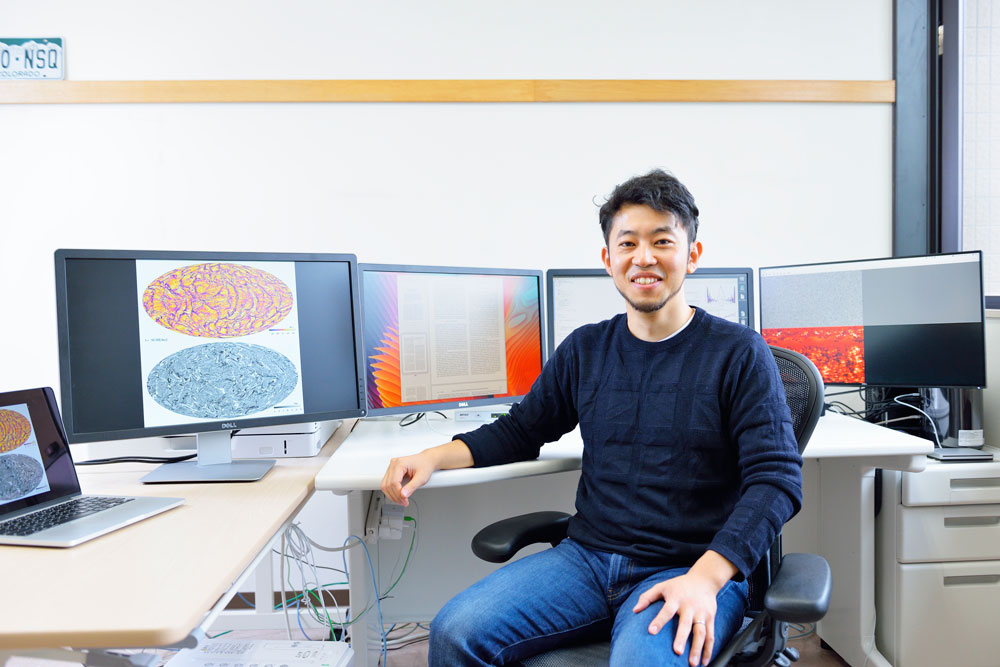
Interviewer: Rue Ikeya
Photographs: Toshiyuki Kono unless noted otherwise
Released on: Dec. 10, 2019 (The Japanese version released on Dec. 10, 2018)